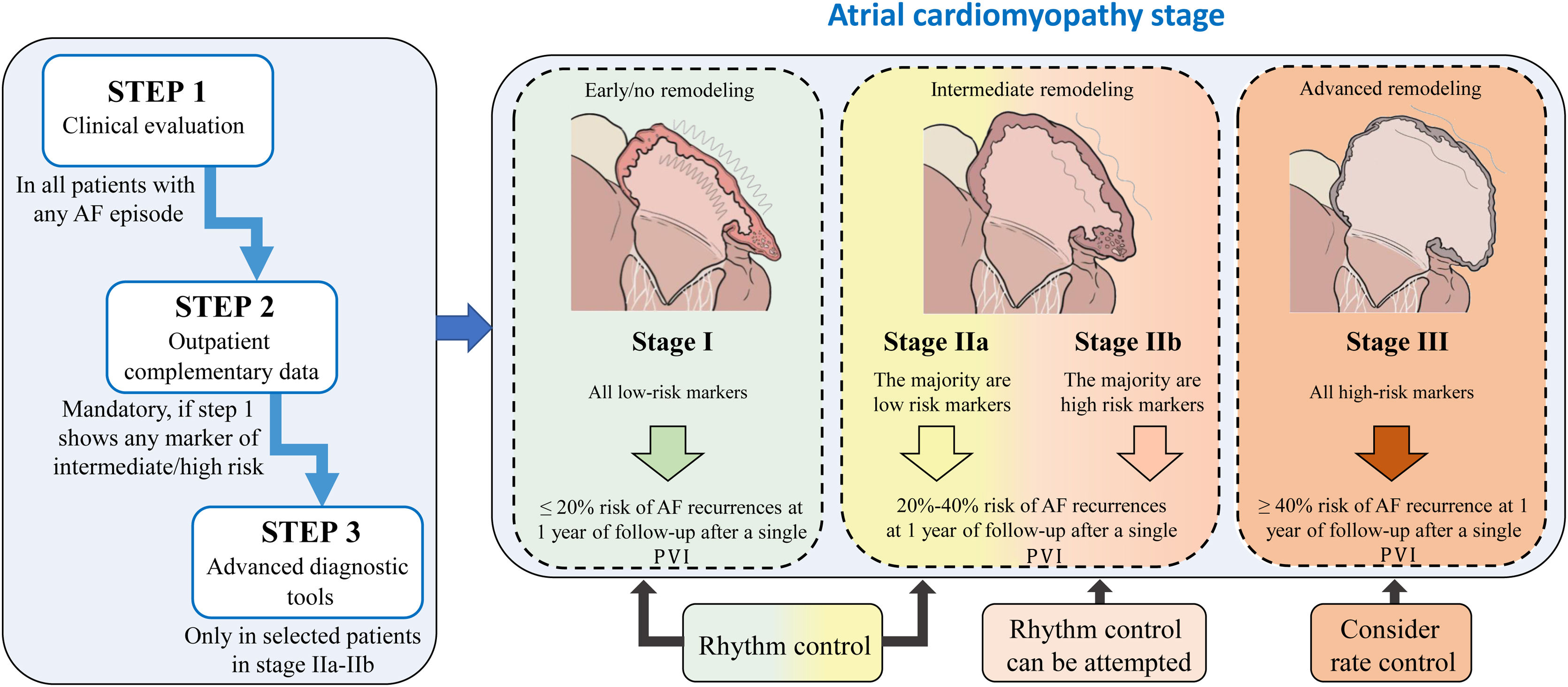
Atrial fibrillation (AF) causes progressive structural and electrical changes in the atria that can be summarized within the general concept of atrial remodeling. In parallel, other clinical characteristics and comorbidities may also affect atrial tissue properties and make the atria susceptible to AF initiation and its long-term persistence. Overall, pathological atrial changes lead to atrial cardiomyopathy with important implications for rhythm control. Although there is general agreement on the role of the atrial substrate for successful rhythm control in AF, the current classification oversimplifies clinical management. The classification uses temporal criteria and does not establish a well-defined strategy to characterize the individual-specific degree of atrial cardiomyopathy. Better characterization of atrial cardiomyopathy may improve the decision-making process on the most appropriate therapeutic option. We review current scientific evidence and propose a practical characterization of the atrial substrate based on 3 evaluation steps starting with a clinical evaluation (step 1), then assess outpatient complementary data (step 2), and finally include information from advanced diagnostic tools (step 3). The information from each of the steps or a combination thereof can be used to classify AF patients in 4 stages of atrial cardiomyopathy, which we also use to estimate the success on effective rhythm control.
Keywords
Atrial fibrillation (AF) represents a growing health burden worldwide, which is increasingly relevant in the current context of limited therapeutic options for effective long-term rhythm control.1,2 Although part of the problem can be attributed to the limitations of current invasive and pharmacological treatments, a more careful look at AF progression indicates that the degree of the underlying atrial cardiomyopathy may have important implications for the success of rhythm control strategies.3,4
In clinical practice, we have been using a simplistic AF classification based on temporal criteria, which considers a 7-day threshold (ie, persistent AF) as the limit to differentiate episodes with lower success rates when attempting rhythm control.5,6 Moreover, after >1 year in AF, the probability of achieving successful rhythm control further decreases,7 highlighting the practical value of the current classification for AF management.5 The most recent guidelines recognize AF as a disease continuum requiring a variety of strategies at different stages and also including patients at risk of AF.8 However, in AF patients, episode duration still remains the main classification criterion, and very limited information is provided on the role of the broad underlying range of remodeling changes during AF progression, which also affect rhythm control.4,9 This is especially important during the first few months of persistent AF episodes when patients are not necessarily in the same underlying remodeling stage despite the same duration of AF.4 In patients with paroxysmal AF episodes, the underlying atrial cardiomyopathy associated with other comorbidities may also predispose some patients to a low probability of successful rhythm control.10 Therefore, a new classification of AF that includes the underlying degree of atrial cardiomyopathy, whether associated with AF remodeling or other comorbidities, will provide a more personalized assessment of the substrate, aiding clinical decision-making and appropriate selection of patients candidates for rhythm control therapies.
Steps in the above direction have been taken with the 4S-AF scheme, which includes atrial substrate assessment in 1 of the 4 categories of the scheme.11 This is in line with current guideline recommendations for a holistic or integrated care approach to AF management,5 whereby adherence to the Atrial fibrillation Better Care pathway is associated with improved clinical outcomes.12 However, the structured characterization of the 4S-AF scheme does not include the evidence supporting the wide variety of comorbidities and cardiovascular risk factors potentially affecting the underlying atrial substrate. Atrial substrate characterization requires well-designed integration of electrical, structural and associated fibrotic remodeling to provide a more complete assessment of the underlying atrial cardiomyopathy.
In this review, we propose an evidence-based practical characterization of the atrial substrate of AF patients with implications for rhythm control. The characterization is based on 3 steps that start with a basic clinical evaluation (step 1), followed by assessment of the outpatient complementary data (step 2) commonly available in outpatient clinics, and finally the inclusion of more advanced information (step 3) that may be available in specialized centers. Each step can be used as a stand-alone approach or in combination with the other 2 steps, thereby enabling physicians working in different clinical settings to obtain an evidence-based assessment of the atrial cardiomyopathy of AF patients. Specifically, we propose the use of these 3 characterization steps to classify AF patients in 4 stages of atrial cardiomyopathy that can be used to estimate the success of active rhythm control.
The association between the variables and parameters included in the characterization steps and the pathological atrial substrate was primarily defined by the scientific evidence on their relationship with atrial fibrosis, low-voltage areas in invasive electroanatomical maps or late gadolinium enhanced (LGE) regions in cardiac magnetic resonance (CMR) imaging. When no evidence from randomized trials or meta-analyses was available, we used prospective series or large (> 100 patients) retrospective registries.
ATRIAL CARDIOMYOPATHY AND ITS CLINICAL IMPLICATIONS FOR AF CLASSIFICATIONAF is both the cause and consequence of morphological and functional alterations of atrial tissue that increase the risk of long-term perpetuation of the arrhythmia.3,4,10 These changes, which are defined as atrial remodeling—including Ca2+ handling abnormalities, action potential duration shortening, atrial fibrosis and autonomic nervous system dysregulation— generate a heterogeneous atrial substrate and make the atria susceptible to AF.10 Although not all remodeling changes are associated with atrial cardiomyopathy, the presence of extensive remodeling and fibrosis increases the risk of AF initiation and recurrences.3,13 Epicardial adipose tissue also contributes to fibrotic remodeling of the atrial myocardium by secreting several adipokines and favoring the fibro-fatty infiltration of subepicardial layers of the atrial wall.14 Furthermore, various cardiac conditions and risk factors may lead to a baseline state of atrial cardiomyopathy.
Importantly, atrial fibrosis is generally accepted as an irreversible indicator of progressive atrial cardiomyopathy,4 which can be used as an objective marker for substrate characterization.3,13 Although histopathological analysis represents the gold standard for the identification and quantification of fibrosis, the availability of human atrial samples for fibrosis assessment and further correlation with clinical variables is rarely found in clinical series.3 However, other clinical tools such as minimally invasive electroanatomical maps and noninvasive CMR imaging have been validated to identify surrogate markers of atrial fibrosis.3,15,16 Therefore, in the present article we have included atrial fibrosis as the primary reference due to its association with the variables included in the characterization steps. Alternatively, we used low-voltage values or LGE-CMR imaging data as surrogates of the underlying fibrotic substrate.
STEP 1: CLINICAL EVALUATIONCardiovascular risk factors and comorbiditiesThe incidence of AF increases with age in both men and women, with a higher incidence in men than in women, However, in patients older than 75 years, 60% of AF patients are women.1,5 Interestingly, the increase in AF risk with age is associated with a linear trend of progressively larger left atrial (LA) regions with LGE on CMR images.17 The latter might explain at least some of the results of a recent subanalysis of the CABANA trial, which has shown progressively higher rates of 4-year AF recurrences after catheter-based ablation or antiarrhythmic drugs in population subsets aged<65 years vs those aged >75 years.18 Although aging is associated with higher levels of underlying atrial fibrosis and higher AF incidence, biological age rather than a specific age cutoff may be more important for determining the risk of AF recurrences and complications.19 Therefore, combining biological age with other variables should help physicians to identify the relevance of age in patient-specific substrates.20
Diabetes mellitus is a well-established risk factor for AF. Diabetes favors a proarrhythmic milieu through various mechanisms, including hyperinsulinemia, chronic inflammation, and activation of profibrotic pathways. Diabetes has been demonstrated to be an independent predictor of fibrotic areas in LGE-CMR images of patients with AF.13 Several prospective studies have confirmed diabetes as an independent predictor of AF recurrences after catheter ablation.21,22 Indeed, glycated hemoglobin (ie, HbA1c) values represent a biomarker of diabetes status with practical clinical implications for cardiovascular risk stratification.23 Chao et al.,22 have shown that patients with normal glucose metabolism (HbA1c=5.5±0.5%) have higher bipolar voltage values than patients with abnormal glucose metabolism (HbA1c=6.2±1.3%). Further evidence from a meta-analysis reported that a 1-point increase in baseline glycated hemoglobin (ranging from 6.04%-7.96%) increases the odds ratio of AF relapse by 0.5 during long-term follow-up after catheter ablation.24 The same meta-analysis showed that the increase in body mass index (BMI) has an independent predictive value for AF recurrences after catheter ablation. Indeed, obesity is directly related to indicators of atrial fibrosis with larger low-voltage areas in obese patients than in lean patients.25 More specifically, patients with BMI values of 30.2±2.6kg/m2 show an increase in low-voltage atrial areas compared with a reference group with a BMI of 25.2±1.3kg/m2.
Hypertension is associated with an increased AF incidence and significant atrial electrical and structural remodeling, characterized by a higher prevalence of slow conduction regions and low-voltage areas in electroanatomical maps.3,26 This is consistent with higher rates of AF recurrence after catheter ablation in patients with uncontrolled hypertension compared with patients without hypertension or controlled hypertension.27 Additional results from a randomized trial evaluating the efficacy of aggressive blood pressure treatment on AF recurrences after catheter ablation indicate that this strategy is not superior to a standard blood pressure control target (< 140/90mm Hg) to decrease AF recurrences.28
The role of other classic cardiovascular risk factors such as dyslipidemia on AF pathophysiology remains unconclusive. Although LDL-cholesterol promotes proinflammatory pathways potentially implicated in AF remodeling, several groups have shown an inverse relationship between total cholesterol and LDL-cholesterol levels and both incident AF and recurrences after catheter ablation.29
The combination of risk factors for metabolic syndrome has proven to be an independent predictor of low-voltage areas on atrial electroanatomical maps.30 In fact, metabolic syndrome is also associated with a higher risk of AF recurrences after catheter-based ablation.31
Obstructive sleep apnea (OSA) has been associated with structural atrial changes and conduction abnormalities that make the atria susceptible to AF.32 However, OSA is often present in AF patients with other comorbidities and the relative contribution of each condition is unclear. Moreover, the association of OSA with AF recurrences after catheter ablation remains unproven. A meta-analysis mainly including retrospective series suggested that OSA is associated with AF recurrences after catheter ablation.33 However, the single randomized study performed to date has not shown significant differences in AF recurrences between OSA patients treated with continuous positive airway pressure and those with standard care after ablation.34 Moreover, the authors of that study also found no differences in AF recurrences between patients with and without OSA.34
Atrial fibrillation presentationA clinical variable that is relatively easy to obtain during a medical interview is the time in AF. The transition from paroxysmal to persistent and long-standing persistent AF is characterized by a progressive increase in atrial structural remodeling and worsening of the atrial cardiomyopathy.35 However, atrial remodeling during AF follows patient-specific patterns and, therefore, the mere fact of having episodes lasting ≥ 7 days does not mean that all patients will be at the same remodeling stage.4,9 Notwithstanding, the longer the time in AF, the higher the probability of more advanced atrial remodeling stages and atrial cardiomyopathy.3,4 A recent study has shown that patients with long-standing persistent AF have significantly higher levels of fibrosis in atrial biopsy samples than patients with paroxysmal and persistent AF episodes.3 The latter suggests that patients with paroxysmal and persistent AF episodes, before reaching the criterion for long-standing persistent AF, show a wide range of patient-specific atrial remodeling patterns with significantly different underlying atrial substrates. This is further highlighted in clinical and experimental series, in which 75% of individuals complete atrial electrical remodeling within a 6-month window of uninterrupted AF episodes (in patients) or high-burden (> 99%) paroxysmal AF episodes (in animals).4,9 This electrical remodeling is reflected in faster electrical activation rates on intracardiac recordings compared with early stages of electrical remodeling. Indeed, electrical remodeling has a prognostic impact in rhythm control.6,9 Therefore, 6 months seems to be a time-dependent threshold that may differentiate early from late remodeling stages associated with AF.
Evidence supporting the implications of the time in AF for successful rhythm control can be obtained from the EAST-AFNET 4 trial and subsequent subanalyses.36,37 The data showed that 56% of the patients who were in persistent AF at baseline in the early rhythm arm of the EAST-AFNET 4 study were in sinus rhythm at 24 months of follow-up. Conversely, only 20% of the patients who were in persistent AF at baseline in the usual care arm were in sinus rhythm at the same follow-up.37 Interestingly, persistent AF patients had been diagnosed on average 77 days earlier, which suggests that the atria may have been in the early stages of AF-related remodeling.4
A meta-analysis of 6 observational studies has revealed that the time between AF diagnosis and first catheter-based ablation is a significant marker of effective rhythm control during follow-up. A diagnosis-to-ablation time ≤ 1 year was associated with a 27% lower relative risk of AF recurrence compared with a diagnosis-to-ablation time >1 year.7 Although these studies do not specifically address the time in AF, the data further highlight that AF itself may lead to advanced stages of atrial cardiomyopathy.
Structural heart diseaseSeveral heart diseases also affect the atrial structural and electrophysiological substrate.3,38 However, the evidence does not support a higher risk of AF recurrences after attempting a rhythm control strategy that may include catheter ablation in all of them. Such is the case for chronic coronary artery disease, for which various series have yielded discordant results in terms of outcomes of catheter ablation to control AF recurrences.39,40
Among other cardiomyopathies, valvular heart disease with at least moderate dysfunction, and in particular, mitral valve regurgitation and mitral stenosis have been shown to significantly affect the atrial substrate.41,42 Valvular heart disease (mainly affecting the mitral and tricuspid valves) has also been associated with a higher risk of AF recurrences after catheter ablation.43,44 Furthermore, a higher risk of AF recurrences has been reported in patients with mitral valve replacement undergoing catheter ablation for AF.42
There is better general agreement on the impact of hypertrophic cardiomyopathy on the development of AF and the risk of recurrences. In fact, AF is the most common arrhythmia in patients with hypertrophic cardiomyopathy with a prevalence of 22.4%.45 Atrial electroanatomical maps in patients with hypertrophic cardiomyopathy and AF show a greater extent of abnormal atrial substrate, as evidenced by low-voltage areas compared with controls who also have AF.46 A recent meta-analysis also reported a higher rate of AF recurrences after a single ablation procedure in patients with hypertrophic cardiomyopathy compared with individuals without the disease.47
Using a more general perspective within the definition of heart failure (HF),48 several series have shown that both symptomatic HF with reduced ejection fraction and preserved ejection fraction are associated with a higher underlying degree of atrial fibrosis and low-voltage areas compared with controls.3,38 It is more difficult to establish the role of HF with mildly reduced ejection fraction, although in symptomatic cases the atrial substrate may also be affected.49 Multicenter data showed that HF patients who had been symptomatic at some stage have a higher risk of AF recurrences after catheter ablation than patients without HF.50
Step 1 is summarized in figure 1.
The degree of atrial cardiomyopathy is based on the evidence supporting the relationship between each clinical variable and the underlying atrial fibrosis or its surrogates. The medical information necessary for this step should be feasible in any conventional clinical visit. Normal glucose metabolism: glycated hemoglobin (HbA1c) ≤ 5.5%; mild/overt abnormal glucose metabolism: HbA1c: 5.5-6.2%/≥ 6.2%. AF, atrial fibrillation; BMI, body mass index; d, days; D/SBP, diastolic/systolic blood pressure; HFr/mr/pEF, heart failure with reduced/mildly reduced/preserved ejection fraction (EF ≤ 40%/EF=41-49%/EF ≥ 50%); mo, months; VHD, valvular heart disease; Y, year.
In this second step, we propose using additional parameters commonly available in outpatient clinics.
Electrocardiographic parametersECG parameters are not routinely implemented in clinical practice, probably because of their limited specificity. Nevertheless, some of them are easy to measure in regular outpatient visits and their value may increase in the context of the entire set of complementary data. Among them, PR interval prolongation in sinus rhythm has been associated with increased AF incidence in a large community-based study with long-term follow-up.51 In a prospective study of patients with AF, PR interval prolongation has also been associated with decreased mean LA low-voltage and increased AF recurrences after catheter ablation. In the same study, AF recurrences were documented in 14% of patients within the first quartile of PR interval values (< 166ms) compared with 22% in patients within the fourth quartile (PR ≥ 202ms).52
Jadidi et al.53 described a method to measure P-wave duration more accurately during sinus rhythm based on digitally amplified signals (40-to-50mm/mV). This approach showed good correlation values with LA low-voltage substrate on electroanatomical maps of patients undergoing AF ablation. In another series, P-wave duration was further demonstrated to be an independent predictor of LA low-voltage substrate and AF recurrences after pulmonary vein isolation (PVI).54 Specifically, a 3-fold higher risk of AF recurrences was estimated in patients with amplified P-wave duration >160ms compared with values <145ms.54 P-wave duration also predicted low-voltage substrate in patients with paroxysmal AF, which further emphasizes the relevance of characterizing the arrhythmogenic atrial substrate rather than using a classification based solely on episode duration.
Blood biomarkersBlood biomarkers of fibrosis, inflammation, hemodynamic overload, and oxidative stress can help to improve risk stratification of AF. In particular, high-sensitivity cardiac troponin-T (hs-cTnT) has been proven to be an independent predictor of all-cause mortality, AF incidence, and cardiovascular mortality.55,56 In a retrospective study of patients undergoing a first AF ablation procedure, Kornej J. et al.57 showed that serum levels of hs-cTnT before ablation were an independent predictor of LA low-voltage areas. In another prospective cohort of 125 consecutive patients undergoing a first ablation procedure, the authors showed that plasma levels of hs-cTnT were significantly higher in patients with recurrences than in those without recurrences (11.7±8.3 pg/mL vs 8.4±4.7 pg/mL).58 The prognostic relevance of hs-cTnT, but not of troponin I, has been further confirmed in a meta-analysis including 27 studies.59
Natriuretic peptides, including the B-type natriuretic peptide and the N-terminal peptide pro-BNP (NT-proBNP), are neurohormones secreted from cardiomyocytes in response to an increase in wall tension from pressure or volume overload. In AF, atrial dilation is a common feature of remodeling and in some subsets with specific underlying cardiac conditions the atria may also be subjected to pressure overload.4,37,60 Clinical data using 3-day Holter monitoring before blood sampling have shown that levels of both mid-regional pro-peptide of atrial natriuretic peptide and NT-proBNP significantly differ among 3 categories of AF/atrial tachycardia burden (0%, 0%-99% and 99%-100%).61 More recently, Rossi et al.62 have described a statistically significant association of NT-pro-BNP levels with low-voltage areas on invasive electroanatomical maps of patients undergoing PVI. Moreover, a cutoff level of serum NT-proBNP >400 ng/L provided a 59% sensitivity and 65% specificity for predicting low-voltage-areas ≥ 20% of the LA surface.62
NT-proBNP levels have also been associated with AF recurrences after catheter ablation in several studies from different groups.63,64 The results were confirmed in recent meta-analyses.63,65 In a prospective study of patients undergoing AF ablation, plasma levels of NT-proBNP ≥291 pg/mL in paroxysmal AF and ≥ 368 pg/mL in persistent AF patients provided the highest sensitivity and specificity for discrimination between patients with and without AF recurrences during a 12-month follow-up.64 Overall, data on NT-proBNP suggest that this biomarker may be a good indicator of the underlying atrial remodeling and also predicts a higher risk of recurrences after catheter ablation.
Among the inflammatory biomarkers, C-reactive protein is widely used in clinical practice in various pathological scenarios. C-reactive protein is an independent predictor of the development of future AF,66 which may be related to a greater degree of LA fibrosis and structural remodeling in the presence of increased levels of C-reactive protein.67 In a recent large multicenter prospective study of 711 patients focusing on the association between C-reactive protein and long-term AF recurrence following catheter ablation, patients in the highest C-reactive protein quartile (> 2.7mg/L) had a 60% higher risk of recurrences than those in the lowest (< 0.8mg/L) after adjustment for known risk factors for recurrence.88 These results have been reproducible across several observational studies and a recent meta-analysis, which lends further support to the role of this biomarker in AF.65
Based on the relationship between structural remodeling and AF progression,4 several biomarkers associated with fibrosis-promoting pathways and extracellular matrix turnover have been studied as potential indicators of the AF stage and predictors of clinical outcomes. In particular, in patients with hypertension and HF undergoing PVI, serum biomarkers of collagen cross-linking and collagen type I deposition have been associated with AF prevalence, incidence and further recurrences during follow-up.68 However, tissue validation of serum biomarkers of collagen cross-linking correlates poorly with the amount of nonsoluble collagen fibers in myocardial samples.69 Moreover, other studies have not shown a significant correlation between blood biomarkers of fibrosis with low-voltage atrial areas in patients undergoing intracardiac mapping.70 Similar negative results have been reported for the correlation of blood biomarkers of fibrosis and the histopathological data in the left and right atrial appendages of patients undergoing cardiac surgery.71
In translational research series, galectin-3 has also shown significant value to predict clinical outcomes in patients with AF. Patients with persistent AF show increased levels of galectin-3 in plasma, and the biomarker is an independent predictor of interstitial fibrosis in right atrial appendage samples from patients undergoing cardiac surgery.72 Some series have also reported that galectin-3 levels are higher in patients with AF recurrences after catheter ablation than in those without recurrences, especially in persistent AF.73–75 Plasma levels of growth differentiation factor-15 (GDF-15), a distant member of the transforming growth factor-β cytokine family, is also associated with clinical outcomes in patients with AF.76 Moreover, GDF-15 levels have also been identified as an independent predictor of AF recurrences after catheter ablation. In particular, a recent prospective study has shown that preprocedural serum GDF-15 values >1287.3 ng/L were associated with an increased rate of AF recurrences after PVI.77 However, GDF-15 is not commonly available in routine blood testing. Less clear is the role of other biomarkers like soluble ST2, a member of the interleukin-1 receptor family.78
In summary, some studies have demonstrated a relevant role of biomarkers in assessing the underlying atrial substrate, which indeed provides further prognostic value. Biomarker data should be interpreted individually based on the specific clinical context of each patient.
Echocardiographic parametersTransthoracic echocardiography allows quantitative assessment of LA diameters, volume, and function, along with indirect assessment of LA stiffness and pressure. This makes the echocardiogram a valuable noninvasive tool to study atrial cardiomyopathy.79
Several meta-analyses have recently shown that a larger indexed LA volume (LAVi) is significantly associated with a higher risk of major cardiovascular events in AF and with a higher risk of AF recurrence after catheter ablation.80,81 LA diameter ≥ 50mm was also identified as an independent predictor of AF recurrence. Nonetheless, relying exclusively on LA diameters may represent a rough estimate of the actual LA size, particularly for the dilated and asymmetrically remodeled atria.79 Therefore, we propose using LAVi on transthoracic echocardiography studies, which provides a more accurate assessment of the 3D structure of the LA. Indeed, LAVi is significantly associated with the extent of enhanced regions in the LA on LGE-CMR images (48±18mL/m2 in stages with <10% of LGE on the atrial wall volume, and 64±24mL/m2 in stages with >30% of LGE on the atrial wall volume).13 Moreover, similar LAVi values in the lower and upper range (50±13mL/m2 and 64±19mL/m2) have been also associated with LA low-voltage atrial areas of 0% and >15%, respectively, in invasive mapping studies.82
Functional echocardiographic indices show a significant association with the underlying substrate. Among functional parameters, the global peak atrial longitudinal strain on speckle tracking echocardiography shows a negative correlation with the grade of LA myocardial fibrosis in samples from patients with severe mitral regurgitation referred for cardiac surgery.83 Moreover, global peak atrial longitudinal strain and LAVi have been described as independent predictors of the extent of LA low-voltage areas in persistent AF patients undergoing catheter-based ablation.82 More specifically, patients without low-voltage atrial areas showed a mean global peak atrial longitudinal strain significantly higher than those with >15% of low-voltage areas in the LA (11.3±5.3% vs 6.2±2.9%, respectively). The same parameters have also been reported as independent predictors of AF recurrences after 9 months of follow-up.82 Step 2 is summarized in figure 2.
The degree of atrial cardiomyopathy is based on the evidence supporting the relationship between each parameter and the underlying atrial fibrosis or its surrogates. The number of available biomarkers, and electrocardiographic and transthoracic echocardiography parameters may vary among different clinical settings. The latter does not preclude the use of the available parameters for characterizing the underlying atrial substrate. CRP, C-reactive protein; Hs-cTnT, high-sensitivity cardiac troponin-T; LAVi, LA volume index; LAd, Left atrial diameter; NT-proBNP, N-terminal pro-brain natriuretic peptide; Px/PsAF, paroxysmal/persistent atrial fibrillation. SR, sinus rhythm.
* LAd is not recommended when LAVi is available.
Atrial fibrosis as a marker of atrial cardiomyopathy can be further assessed with advanced diagnostic tools. Such tools are not routinely available in primary care centers or outpatient clinics, and can usually only be performed in referral centers for AF management, in which a more personalized treatment strategy may be possible.
The LA stiffness index (the ratio of E/e’ to the global peak atrial longitudinal strain) is a novel echocardiography-based parameter with independent predictive value of LA low-voltage areas in persistent AF patients undergoing catheter-based ablation.82 The LA stiffness index was superior to the global peak atrial longitudinal strain and LAVi in predicting low-voltage areas in the LA.82 This index is also an independent predictor of AF recurrences after ablation.
Recently, Enríquez-Vázquez et al.4 reported a noninvasive and practical parameter that may enable physicians to assess the underlying atrial remodeling stage in patients with AF. These authors showed that simultaneous atrial electromechanical assessment during AF identifies early atrial remodeling changes with clinical impact in short- and long-term rhythm control. More specifically, they demonstrated that during AF the atrial electrical activation rates may be faster than the simultaneously recorded mechanical contractions. This phenomenon was defined as atrial electromechanical dissociation, which indeed was demonstrated to be an early indicator of the individual-specific atrial remodeling stage in patients with AF episodes lasting ≤ 6 months. This new parameter showed higher predictive performance for successful rhythm control than conventional echocardiography parameters or blood biomarkers in patients at early stages of atrial remodeling progression.
LA fibrosis is associated with new-onset AF, perpetuation of AF, and recurrences after catheter ablation.3,4,10,17 On LGE-CMR images, it is possible to noninvasively assess areas with increased extracellular matrix, the hallmark of atrial fibrosis, which correlated with the gold standard histological analysis.13,84 Marrouche et al.85 have reported an unadjusted overall hazard ratio of 1.06 per 1% increase in atrial fibrosis based on LGE-CMR images of the LA. Similar results were obtained after covariate adjustment. In fact, addition of LGE-CMR-based fibrosis quantification improved the predictive accuracy of recurrences using a model with traditional clinical covariates.85 Similarly, Quail et al.86 described a 2-fold increased risk of new-onset atrial arrhythmias for each 1 standard deviation increase of enhanced values on LGE-CMR images.
However, hyperenhancement on CMR images is not exclusively related to fibrosis or to a specific type of fibrosis. The latter makes it challenging to differentiate between hyperenhanced areas caused by replacement fibrosis, iatrogenic scar or physiological extracellular matrix, and areas of further interference with adjacent hyperenhanced structures, such as epicardial fat. Moreover, the signal intensity threshold to discriminate between normal and fibrotic atrial tissue is not homogeneous among series, which makes scar assessment sensitive to bias.16 Additionally, high-quality imaging acquisition with high spatial resolution is crucial for accurate noninvasive assessment of atrial fibrosis. Normalization of LGE to the blood pool is also an important step in minimizing interpatient variability, as it corrects for patient-specific pharmacokinetics of the contrast agent. LGE-CMR imaging is also sensitive to artifacts caused by the respiratory navigator restore pulse, which results in higher signal intensity in the tissue around the navigator (eg, the neighboring atrial wall or the blood pool).87
Invasive electroanatomical mapping of the atria enables cardiac electrophysiologists to detect low-voltage regions, which represents an alternative approach to assess the atrial fibrotic substrate. In addition to fibrosis, increased intercellular space, myofibrillar loss, and decreased myocardial nuclear density are also significantly associated with voltage reduction and slow conduction zones.3 Indeed, several series have described a proportional reduction in bipolar voltage with each unit of increase in local image intensity ratio on LGE-CMR images of the LA. This ratio was computed as the mean myocardial signal intensity in the LA divided by the mean signal intensity in the blood pool.88,89 Zghaib et al.89 have reported that an image intensity ratio >0.74 corresponds to a bipolar voltage <0.5mV. Khurram et al.88 reported a slightly higher image intensity ratio (> 0.97) for the same bipolar voltage values <0.5mV.
Multiple studies also support the prognostic value of assessing the extent of low-voltage atrial areas during invasive electroanatomical mapping.90,91 Recent data show that the extent of low-voltage values in paroxysmal and persistent AF (considering low-voltage as <0.5mV in sinus rhythm or <0.24mV in AF) may show a different risk of recurrences after ablation.91 More specifically, low-voltage areas affecting >5% of the LA surface increase the risk of AF recurrences in paroxysmal AF, although in persistent AF this predictive low-voltage threshold has been observed with areas affecting >15% of LA surface.91 This might suggest that in paroxysmal AF low-voltage areas represent a marker of an underlying diffuse atrial cardiomyopathy. Conversely, in persistent AF, low-voltage may be partially caused by reversible remodeling enhanced by the AF itself. However, voltage maps are not free of limitations and potential bias. Thus, bipolar voltage values are sensitive to the intrinsic rhythm at the time of mapping, electrode size, interelectrode spacing, catheter orientation, and tissue contact.92
Finally, current biotechnology tools have provided significant advances in defining the genetic architecture of AF patients. Several rare and common genetic variants have been associated with the incidence of AF and long-term outcomes. The heritability of AF has been reported to be as high as 62% in twins and 22% in the general population. Several groups have investigated the association of AF-related single nucleotide polymorphisms with successful rhythm control after catheter ablation. The most significant polymorphisms associated with AF recurrence after catheter ablation are those on chromosome 4q25 affecting the PITX2 gene.93 However, recent findings from genome-wide association studies involving large patient cohorts do not provide conclusive evidence regarding the ability to predict AF recurrences after catheter ablation based on genetic variants or single nucleotide polymorphisms at the PITX2 locus.94,95 Step 3 is summarized in figure 3.
The degree of atrial cardiomyopathy is based on the evidence supporting the relationship between each parameter and the underlying atrial fibrosis or its surrogates. The number of available parameters may vary among different clinical settings, which does not preclude the use of the available parameters for characterizing the underlying atrial substrate. Utah stages and image intensity ratio are assessed on 3-dimensional late gadolinium enhancement cardiac magnetic resonance images of the left atrium. AF, atrial fibrillation; EMD, electromechanical dissociation; LAVi, left atrial volume index; TTE, transthoracic echocardiography.
We propose a pragmatic classification of AF patients in 4 stages of atrial cardiomyopathy based on the available clinical data and complementary information obtained from each of the evaluation steps. This will enable physicians working in different clinical settings, from outpatient clinics to specialized units in referral centers, to estimate the underlying degree of atrial cardiomyopathy, which may indeed affect the success of active rhythm control. In fact, the classification shows the expected probability of AF recurrences after 1 year of follow-up within each stage of atrial cardiomyopathy (figure 4). AF recurrences after ablation have been established from series addressing the impact of atrial scar and low-voltage areas, as surrogates of atrial fibrosis, in successful rhythm control after ablation.90,91,96,97 Prediction of AF recurrences after catheter ablation has also been proposed in multiple series using different scores, although in general, there are highly variable results among series with a lack of good performance.98 Of note, we were unable to access to the original databases to analyze the raw data of the variables and parameters we propose to assess the underlying atrial cardiomyopathy. Therefore, we are unable to provide a risk score with the specific contribution of each variable. Consequently, this document should be interpreted as descriptive, to highlight the need for a better classification of AF patients. Nevertheless, the classification proposal is based on relevant scientific evidence for each of the steps.
Central illustration. This classification should be interpreted as descriptive, aiming to highlight the need for a better classification of AF patients. Because of the lack of access to the variables and parameters used to generate the stages, we are unable to provide a risk score with the specific contribution of each variable for a more accurate stratification. AF recurrences after ablation have been estimated from series addressing the impact of atrial scar and low-voltage areas in successful rhythm control after ablation90,91,96,97. AF, atrial fibrillation; PVI: pulmonary vein isolation.
Further prospective series are warranted to demonstrate the clinical value of this classification proposal, not only for rhythm control but also for other clinical outcomes in AF patients. Success rates after PVI may change if multiple catheter ablation procedures or surgical ablation are considered after recurrences. Nevertheless, clinical decisions for rhythm control must be always interpreted in the context of each individual case and clinical context, considering the patient's preferences, the presence of symptoms, and importantly, optimized treatment of concomitant comorbidities and cardiovascular risk factors.5
FUNDINGThis work was supported by the European Union Horizon 2020 research and innovation program under Grant Agreement #965286 and #899871. The study was also supported by the Ministry of Science and Innovation (MCIN) (PID2019-109329RB-I00) funded by MCIN/AEI/10.13039/501100011033 and the Fundación Interhospitalaria para la Investigación Cardiovascular. The Centro Nacional de Investigaciones Cardiovasculares (CNIC) is supported by the Instituto de Salud Carlos III (ISCIII), the Ministerio de Ciencia e Innovación (MCIN) and the Pro CNIC Foundation and is a Severo Ochoa Center of Excellence (funded by MICIN/AEI/10.13039/501100011033). Supported in part by grants (PI18/01268 & PI23/00520) from ISCIII, the MCIN.
DECLARATION ON THE USE OF ARTIFICIAL INTELLIGENCEArtificial intelligence was not used for any of the tasks associated with the preparation of this manuscript.
AUTHORS’ CONTRIBUTIONSD. Filgueiras-Rama, F. Marín, G. La Rosa and L. Sanchis participated in the conceptualization, methodology and writing of the original draft of the manuscript. All authors participated in editing and writing the final version of the manuscript. Funding acquisition was obtained by D. Filgueiras-Rama, F. Marín, L. Sanchis, S.N. Hatem, J. Jalife, N. Pérez-Castellano, D. Calvo, and J. Pérez-Villacastín.
CONFLICTS OF INTERESTD. Filgueiras-Rama and L. Sanchis are associate editors of Rev Esp Cardiol. The journal's editorial procedure to ensure impartial processing of the manuscript has been followed. D. Filgueiras-Rama, J.G. Quintanilla and D. Calvo are inventors of pending patent applications related to advanced tools for cardiac fibrillation assessment.
We thank Carlos Galán-Arriola for his support in designing the atrial cardiomyopathy stages of figure 4.